How PINK1 Activates to Affect Neurons Captured for First Time
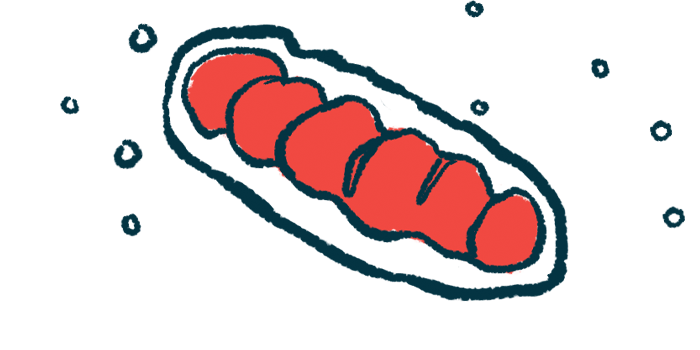
In a “world-first,” scientists in Australia report in detail what happens to the PINK1 protein when it is activated, helping to clear the “confusion” that has surrounded this support protein for cells that include dopamine-producing neurons.
Since mutations in the PINK1 gene that codes for this protein are known to cause some forms of familial Parkinson’s disease, the discovery could open new avenues for treatment.
“Biotech and pharmaceutical companies are already looking at this protein and this pathway as a therapeutic target for Parkinson’s disease, but they have been flying a bit blind. I think they’ll be really excited to see this incredible new structural information that our team has been able to produce,” David Komander, PhD, a professor at Walter and Eliza Hall Institute of Medical Research (WEHI) and the study’s lead author, said in a press release.
The study, “Activation mechanism of PINK1,” was published in the journal Nature in an unedited (but peer-reviewed) version to “give early access to its findings.”
Mitochondria are subcellular structures often called the “powerhouse of the cell” because they are chiefly responsible for making cellular energy. The PINK1 protein plays a critical role in mitophagy, the process that cells use to recycle damaged or dysfunctional mitochondria and replace them with new ones.
When the PINK1 protein is stabilised on the surface of mitochondria and is activated, it sends signals to other cellular components to initiate mitophagy.
In some forms of Parkinson’s, however, mutations in the PINK1 gene lead to a dysfunctional PINK1 protein, which impairs mitophagy so that, ultimately, cells aren’t able to get enough energy to work as intended. This is believed to be a driving force behind nerve cell death in Parkinson’s associated with PINK1 mutations, and mitochondrial dysfunction has been strongly linked to Parkinson’s in general.
Despite the known role of the PINK1 protein in mitophagy, the details of what actually happens when the protein becomes activated — in terms of changes at the molecular level — were unclear.
“Many papers from laboratories around the world — including ours — have captured snapshots of the PINK1 protein. However, the differences in these snapshots has in some ways fueled confusion about the protein and its structure,” said Zhong Yan Gan, a doctoral student at WEHI and the study’s first author.
Gan and colleagues used a new technique called cryogenic electron microscopy (cryo-EM) to observe the PINK1 protein in motion.
“This is the first time that we have used cryo-EM at WEHI to solve the structure of small proteins such as PINK1,” said Alisa Glukhova, PhD, a WEHI professor and study co-author. “This revolutionary technique has only been available in the past five years, and … is a great example of how innovative technologies can really drive forward research and lead to transformative discoveries,” Glukhova added.
The researchers basically used cryo-EM to take pictures of the PINK1 protein at different points in time before, during, and after its activation. Then, these individual snapshots were “stitched together” — sort of like stop-motion animation, according to Gan — to demonstrate the series of events by which PINK1 activates.
“We were then able to reconcile why all these previous structural images were different — they were snapshots taken at different moments in time as this protein was activated to perform its function in the cell,” Gan said. “There are tens of thousands of papers on this protein family, but to visualize how this protein comes together and changes in the process of activation, is really a world-first.”
A notable finding from this work is that, when activated, PINK1 forms a dimer, a pairing of two proteins connected to each other that function in concert. Specifically, the dimer allows PINK1 proteins to attach phosphate groups to themselves — a chemical modification called autophosphorylation that is crucial for their activation.
Forming the dimer is “essential for switching on or activating the protein to perform its function,” Gan said.
Another notable finding is that, even when not in its “active” state, the PINK1 protein still retained kinase activity. In other words, its structure suggests that even the inactive protein can have biochemical effects on other cellular components.
The scientists noted a need for further research to figure out what substance or substances in the cell might interact with PINK1, and the functional consequences of these interactions.
“Our mechanistic insights will likely help efforts to exploit PINK1 as a drug target to stimulate mitophagy and treat” Parkinson’s, the team concluded.