New Bioelectronic Devices May Aid in Monitoring, Treating Parkinson’s
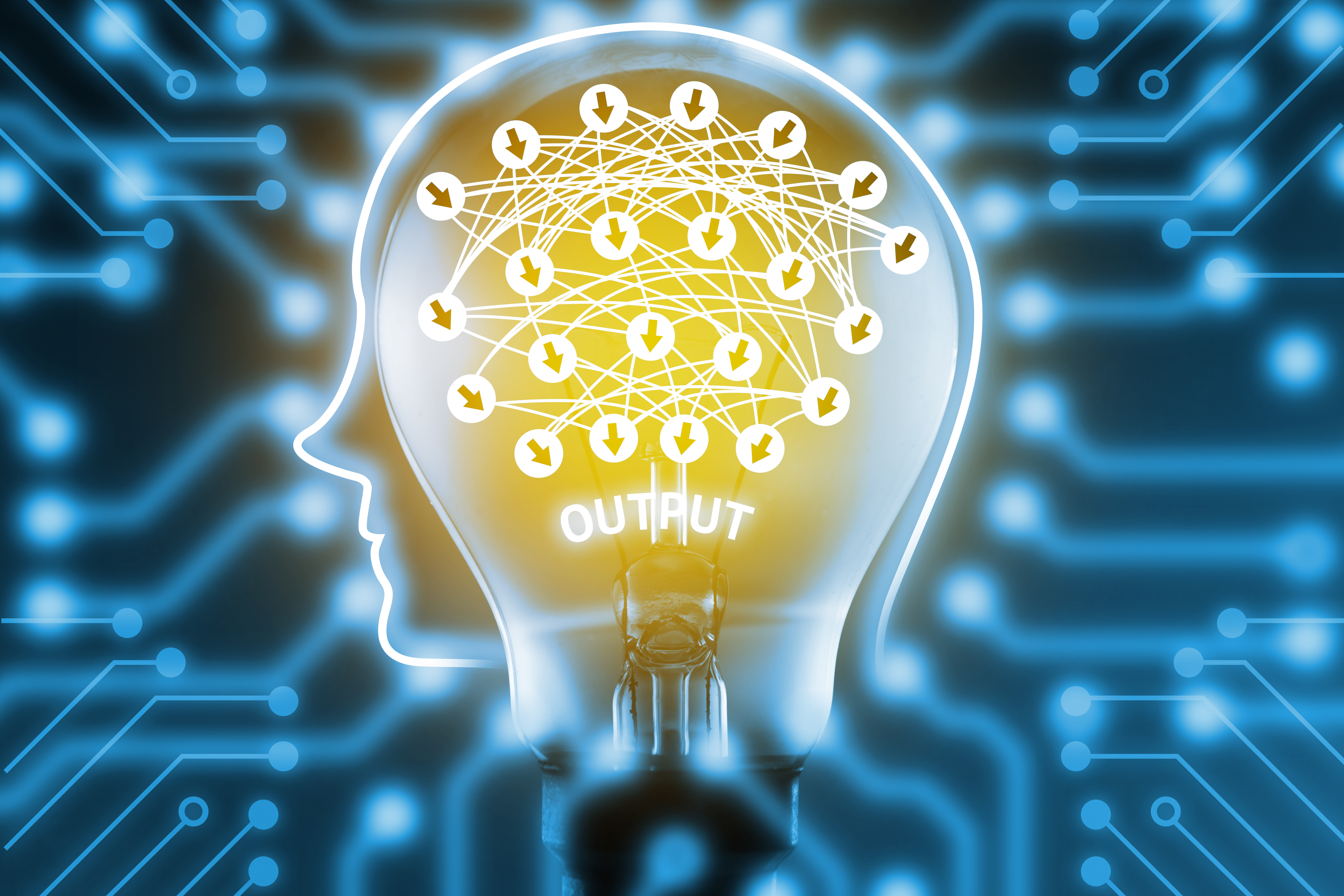
Special bioelectronic devices that can be placed inside a person to diagnose, monitor, and treat neurological disorders, including Parkinson’s disease and epilepsy, may have taken a major step forward with the design of soft and flexible devices that are also biocompatible.
Dion Khodagholy, PhD, assistant professor of electrical engineering at Columbia University, recently published two studies on such new devices that may open the door to new therapies.
“Instead of having large implants encapsulated in thick metal boxes to protect the body and electronics from each other, such as those used in pacemakers, and cochlear and brain implants, we could do so much more if our devices were smaller, flexible, and inherently compatible with our body environment,” Khodagholy, who leads the Translational NeuroElectronics Lab at Columbia Engineering, said in a university news story.
“Over the past several years, my group has been working to use unique properties of materials to develop novel electronic devices that allow efficient interaction with biological substrates — specifically neural networks and the brain,” she added.
In both papers, Khodagholy worked alongside Jennifer N. Gelinas, MD, PhD, an assistant professor of neurology at the Institute for Genomic Medicine at Columbia University Irving Medical Center.
In the first study, “Enhancement-mode ion-based transistor as a comprehensive interface and real-time processing unit for in vivo electrophysiology,” published in the journal Nature Materials, they described the creation of organic transistors designed to record the electrical activity of neurons, and to perform real-time calculations that help in identifying and monitoring neurological disorders.
Transistors are devices that generate, amplify, and control the transmission of electrical signals.
Conventional transistors are made of silicon, and have to be encapsulated in a metal or plastic case when placed inside the body to protect from water and ions (electrically charged atoms). These new transistors are made up of organic materials that allow them to pick up and interact with the ion-driven signals that cells use to communicate.
An initial drawback, however, was that these organic transistors lacked electrical power to record and process brain signals.
To overcome this, Khodagholy and his team created a new version of these transistors with mobile ions embedded into their channels.
The team’s new ion-driven transistors, which they named e-IGTs, were able to capture a wide range of signals, including electrical impulses from neurons.
Investigators then used them as a basis to build soft, biocompatible devices that could be remain inside a person’s body for long periods of time, capturing and recording electrical signals associated with epilepsy.
“We’re excited about these findings,” Gelinas said. “We’ve shown that e-IGTs offer a safe, reliable, and high-performance building block for chronically implanted bioelectronics, and I am optimistic that these devices will enable us to safely expand how we use bioelectronic devices to address neurologic disease.”
In the second study, “Mixed-conducting particulate composites for soft electronics,” published in the journal Science Advances, the team described the creation of a soft mixed-conducting particulate material (MCP) that enabled them to build fully biocompatible devices to record muscle and brain electrical activity during deep brain stimulation (DBS) in patients.
DBS is a surgical procedure that involves implanting a device to stimulate specific regions of the brain with electrical signals generated by a battery. The procedure is approved to treat several neurological conditions, including Parkinson’s, epilepsy, and obsessive-compulsive disorder (OCD).
Using living cells that communicate through electrical signals as an inspiration, the team created MCP, a special material that is able to perform several electronic functions by varying the size and density of its electron-conductive particles.
Because it is fully biocompatible, MCP can also bridge the gap between rigid electronic components and soft body tissues, allowing for more effective electronic bonding.
“This innovation opens the door to a fundamentally different approach to electronic device design, mimicking biological networks and creating multifunctional circuits from purely biodegradable and biocompatible components,” Khodagholy said.
E-IGTs and MCP may support the development of a range of biocompatible and bioelectronic devices for therapeutic applications. Both use inexpensive components and can easily be manufactured at a large scale, their developers said.
Khodagholy and Gelinas are now working to create fully functional devices based on these components, which can be used to record and control brain’s electrical activity in people with Parkinson’s, epilepsy, and other neurological diseases.
“Our ultimate goal is to create accessible bioelectronic devices that can improve peoples’ quality of life, and with these new materials and components, it feels like we have stepped closer to that,” Khodagholy said.