Researchers Explore Neural Interactions Behind Reach-to-grasp Tasks
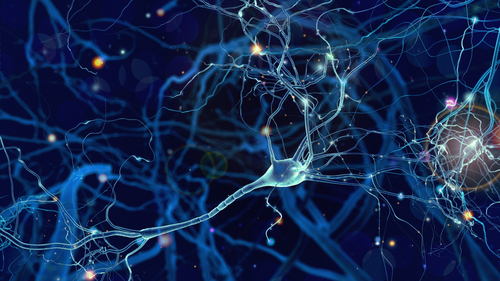
Seemingly simple reach-to-grasp tasks rely on more complex interactions between two specific types of nerve cells in the basal ganglia — the brain region that controls movement and is mainly affected in Parkinson’s disease — than previously thought, a study in mice shows.
The data highlighted that both types of nerve cells, or neurons, are needed for the completion of the movement (being key for different phases of the move), challenging previous studies showing that one type helped initiate the movement, while the other suppressed it.
“This study has helped us understand more about how our brains work and contributes to the body of research that will one day make finding a cure to neurodegenerative diseases possible,” Gordon Arbuthnott, PhD, the study’s senior author, said in a news story. Arbuthnott is the principal investigator of the Brain Mechanisms for Behavior Unit at Okinawa Institute of Science and Technology Graduate University, in Japan.
“With Parkinson’s disease, we’re getting good at fixing the symptoms, but we need to get to the root of the disease,” Arbuthnott added, noting that “in the meantime, understanding how neurons are involved in movement control will help to solve the problems facing patients on a daily basis.”
The study, “Striatal bilateral control of skilled forelimb movement,” was published in the journal Cell Reports.
In people with Parkinson’s, dopamine — one of the major chemical messengers in the brain — is progressively lost in the striatum, a part of the basal ganglia, which is the dopamine-dependent brain region that controls movement.
This results in a gradual loss of coordination and movement that makes seemingly simple daily life tasks, including reaching-and-grasping movements such as picking up a cup of coffee or putting on a jacket, nearly impossible.
Such skilled movements require coordination between both sides of the brain and signals from two neuronal groups in the striatum identified by the presence of D1 (D1 neurons) or D2 (D2 neurons) dopamine receptors in their cell surface.
“We know that interruptions to these basal ganglia neurons are involved in the development of diseases like Parkinson’s and Huntington’s, but the exact cellular mechanisms behind this isn’t properly understood,” said Arbuthnott.
D1 neurons are involved in a “direct pathway” of movement, while D2 neurons are part of the “indirect pathway” that takes a longer route via other areas of the brain. Each behavior or movement relies on a specific group made up of different compositions of these two types.
As such, movement is based on a dynamic system in which these two different types of neurons work together.
While there is still no consensus on the specific roles of these neurons, previous studies have suggested that in movement tasks, the D1 type gives the “go” signal, helping to initiate movement, while D2 neurons give the “stop” signals, suppressing it.
To test this idea, Arbuthnott’s team analyzed how changing the activity of each type of neuron affected a simple reach-to-grasp task in mice.
The task consisted of reaching only one forelimb through an opening in the mouse’s chamber and grasping a chocolate-flavored food pellet. Detailed analyses of mice’s movements through the task revealed that failure resulted from one of three types of errors: initial (about 20% of the misses), final (about 20%), or grasp (about 60%).
An “initial” error was characterized by the mouse’s inability to get its paw through the opening, while a “final” error occurred when the mouse’s paw missed the target after crossing the opening. The “grasp” error was when the mouse’s paw made it to the pellet, but was unable to pick it up.
With this in mind, the researchers assessed whether boosting or suppressing the activity of D1 or D2 neurons in the mice’s brain affected their success rate at the task and the frequency of different types of errors.
Results showed that both activation and suppression of D1 neurons significantly reduced the mice’s success rate, while significantly increasing the number of “initial” errors.
This suggested that either manipulation disrupted the overall dynamics of D1 neurons involved in the task, particularly in the first part of the movement.
In addition, activating the D2 type resulted in a significant drop in the success rate, but this time involving the “final” error. Interestingly, when the team suppressed the activity of D2 neurons, the success rate of treat retrieval increased.
“Our results indicate that [D1 neurons] are clearly involved in the early parts of the reaching movement (trajectory) and that excitation of [D2 neurons] results in disrupted targeting in the later parts of the reach,” the researchers wrote.
Notably, Arbuthnott and his team have developed a theory for the contrasting effects seen in D2 neurons.
“If we imagine that a small group of D2 neurons is enough to reach the target then exciting all the D2 cells in the area might make the small group get lost in the noisy activity of the many, while inhibiting the large group could leave behind just the most strongly excited neurons, which can then effectively guide the mouse’s behavior,” Arbuthnott said.
These findings support a model in which D1 and D2 neurons, and subsequently the “direct” and “indirect” pathways of the basal ganglia, “act together in executing movement and separately in different temporal sequences,” the researchers wrote.
Further analysis also showed that practice of the movement itself changed the connections between neurons in different ways depending on the side of the brain where they were located, compared to the paw used.
Future studies are needed to confirm these findings and proposed models, the team noted.