Neurons Adapt Metabolism to Resist Mitochondria-associated Neurodegeneration in Mice
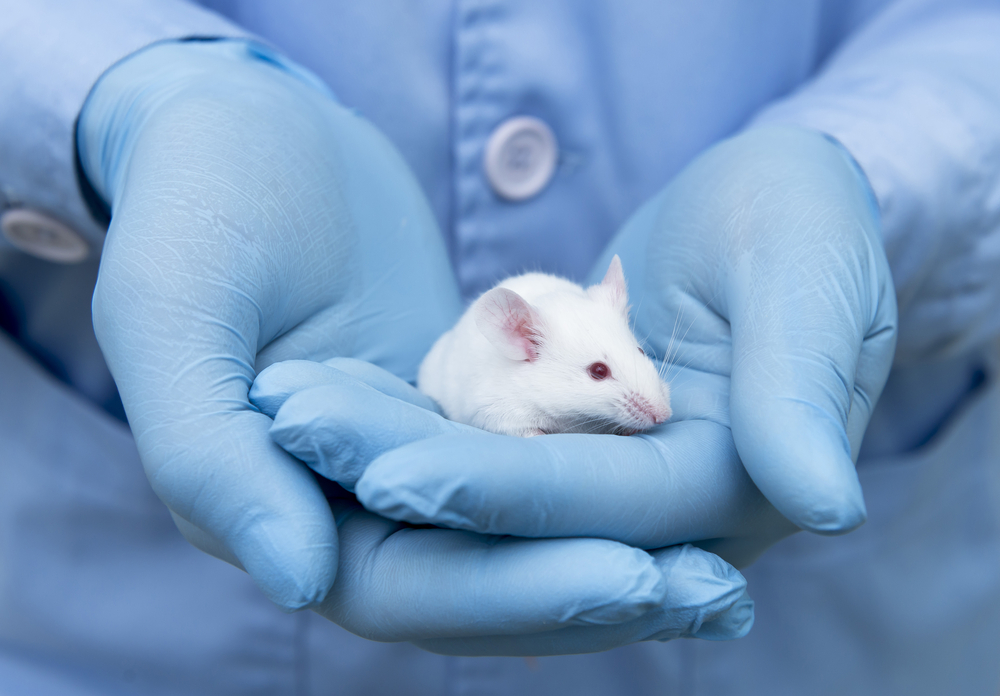
In the presence of mitochondrial dysfunction, nerve cells (neurons) switch to an alternative metabolic program to resist neurodegeneration, according to a recent study in mice.
Interestingly, the data suggested that such a metabolic program is not a one-way road to neurodegeneration, but rather a compensatory mechanism that can be reversed immediately before cell death, effectively preventing it.
These findings contradict the well-established view that neurons cannot adapt their energy metabolism to challenging conditions and irreversibly die, which may open new therapeutic avenues for neurodegenerative disorders associated with mitochondrial dysfunction, such as Parkinson’s disease.
The study, “Neuronal metabolic rewiring promotes resilience to neurodegeneration caused by mitochondrial dysfunction,” was published in the journal Science Advances.
Mitochondria are small organelles inside cells that work as their “powerhouses.” Since nerve cells require large amounts of energy, defects in mitochondria have been linked to several neurodegenerative diseases, including Parkinson’s.
However, the exact mechanisms by which dysfunctional or damaged mitochondria contribute to neuronal death remain largely unclear.
Neurons are generally considered to have no, or very little, capacity to adapt their energy metabolism, and such adaptations are thought to be limited to peripheral tissues and glial cells — whose main function is to support, protect, and repair neurons in the brain and spinal cord.
However, evidence from some neurological diseases suggests that mitochondrial dysfunction can be tolerated for long periods of time, suggesting metabolic adaptation.
Now, researchers at the Karolinska Institutet, in Sweden, and Max Planck Institute for Biology of Ageing, in Germany, set out to evaluate what happens to neurons’ metabolism at different stages of neurodegeneration associated with mitochondrial dysfunction in mice.
They analyzed the metabolism of specific nerve cells of mice genetically altered so that these cells lack the Mfn2 gene (involved in maintaining mitochondrial structure), causing mitochondrial damage and neurodegeneration.
“We devised an innovative approach to purify degenerating neurons from the mouse brain and to analyze the global protein content, the proteome, of these neurons,” Nils-Göran Larsson, MD, PhD, the study’s senior author, said in a news story on the Karolinska website.
“Unexpectedly, the proteomic data showed the existence of a precisely coordinated, neuron-specific metabolic program that becomes activated in response to mitochondrial dysfunction,” added Larsson, who also is a professor at the Department of Medical Biochemistry and Biophysics at Karolinska Institutet.
This metabolic rewiring involved the activation of enzymes implicated in anaplerosis — the process of replenishing depleted metabolic intermediate molecules — which is essential to energy production.
Further analyses supported anaplerosis’ neuroprotective role, as suppression of one of such anaplerotic enzymes resulted in marked acceleration of neuronal death in these mice.
Moreover, and to the researchers’ surprise, reintroduction of the Mfn2 gene in end-stage degenerating neurons was sufficient to reverse key metabolic hallmarks of anaplerosis and prevent their death.
“To our surprise, this whole rewiring process does not necessarily mark terminal metabolic states that gradually and irreversibly accompany neurodegeneration, but our data show that it likely constitutes a compensatory mechanism to sustain neuronal function even at stages immediately preceding cell death,” the researchers wrote.
“Our findings identify a previously unappreciated pathway conferring resilience to mitochondrial dysfunction and show that neurodegeneration can be reversed even at advanced disease stages,” they added.
The discovery of this metabolic rewiring in dysfunctional neurons sheds light on the underlying mechanisms of neurodegeneration and may help to identify new therapeutic approaches to prolong neuronal survival during mitochondrial dysfunction in neurodegenerative diseases.
“Future studies aiming at dissecting the changes in energy metabolism in other brain cell types are required to fully reveal the applicability of this principle to other neurological diseases,” the researchers concluded.