How Enzyme Works to Produce Dopamine in Brain Detailed in Study
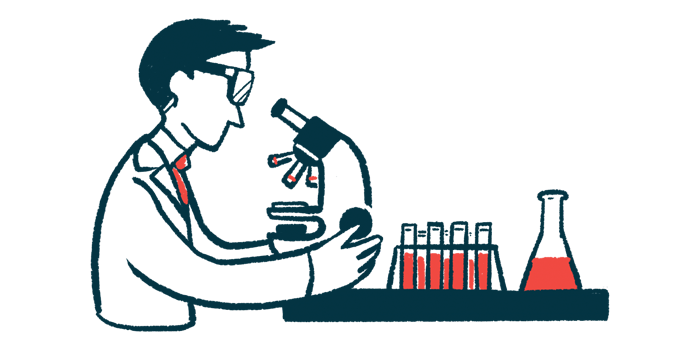
A collaborative effort between researchers in Spain and Norway is helping to enrich an understanding of the regulatory mechanisms behind the production of dopamine, the brain chemical messenger progressively lost in people with Parkinson’s disease.
Specifically, the team determined the structure of tyrosine hydroxylase (TH) — the rate-limiting enzyme in dopamine production — as well as the regulatory mechanisms of its activity, which involve dopamine itself.
“This knowledge gives us new opportunities to develop medicines for neuropsychiatric and neurodegenerative diseases” such as Parkinson’s, Aurora Martinez, PhD, the study’s co-senior author and a professor of biomedicine at the University of Bergen, said in a press release.
Findings were described in the study “Structural mechanism for tyrosine hydroxylase inhibition by dopamine and reactivation by Ser40 phosphorylation,” published in the journal Nature Communications.
Parkinson’s disease is characterized by the progressive loss of dopamine-producing neurons and dopamine signaling in the substantia nigra and the striatum, two brain regions involved in the control of voluntary movement.
In the brain, dopamine production is mainly dependent on the activity of TH, which converts the amino acid tyrosine into dopamine’s precursor L-dopa. L-dopa is then converted into dopamine by an enzyme called dopa decarboxylase.
“A deficiency in striatal TH is a hallmark of Parkinson’s disease,” the researchers wrote, and TH dysfunction leads to dopamine deficiency and parkinsonisms — diseases characterized by Parkinson’s-like symptoms.
As such, understanding the mechanisms that regulate the enzyme’s function may help to identify new treatment targets and approaches for these disorders.
It is well-established that dopamine directly binds to tyrosine hydroxylase, suppressing its activity. This type of regulation, called negative feedback, ensures that dopamine production is ‘turned off’ when cells have sufficient dopamine.
In turn, TH is known to be reactivated by phosphorylation, a process in which a chemical tag, called phosphoryl group, is added to specific regions in proteins and enzymes, regulating their activity.
A lack of insight into TH’s detailed 3D structure, however, has hampered “a deep understanding of the molecular determinants of the interaction between TH … and DA [dopamine],” the researchers wrote.
“Such 3D structures can tell us how the cellular processes take place at the atomic level and thus also how we can find targeted treatment to correct errors in enzymes, which, for example, occur by disease mutations,” Martinez said.
“For TH, such mutations cause TH deficiency, a neurological disease classified as a subgroup of parkinsonism,” she added.
The researchers used state-of-the-art technology, called cryogenic electron microscopy, to determine the 3D structure of TH, both in the absence and presence of dopamine, and with and without phosphorylation.
Among their discoveries, they found that TH’s flexible N-terminal region, which forms a tail-like structure, is key in regulating the enzyme’s activity.
This region is usually free and detached from TH’s main structure when the enzyme is active, results showed. Upon dopamine binding to the enzyme’s so-called active site, tyrosine hydroxylase’s structure changes in a way that the N-terminal region attaches to dopamine and the enzyme’s active site, blocking dopamine’s exit.
This “contributes to the high-affinity binding and strong inhibition [suppression] of TH activity,” the researchers wrote.
They also found that TH phosphorylation prompts the detachment of the N-terminal region from the enzyme’s active site, allowing the release of dopamine and the restoration of TH activity.
“When the dopamine level drops again, signaling pathways will be activated in the cell,” said Rune Kleppe, PhD, a study author with the department of occupational medicine at Haukeland University Hospital, also in Bergen.
“This leads to TH being modified, by so-called phosphorylation, which causes dopamine to be released and the enzyme to be reactivated,” Kleppe added.
These findings highlight “the molecular basis of the inhibiting and stabilizing effects of DA and its counteraction by S40-phosphorylation, key regulatory mechanisms for homeostasis [balance] of DA and TH,” the researchers wrote.
“Cryoelectron microscopy has been essential to find out what TH looks like and has also made it possible to see how it changes when it binds dopamine,” Martinez said.
The “structural knowledge” gained through this work “allows for a more detailed mechanistic understanding of key physiological regulation of TH,” the researchers wrote. This may help in the development of new therapies for dopamine-related neurological diseases, such as Parkinson’s and parkinsonisms.
This work was a combination of long-term research into TH activity by scientists at the University of Bergen and Haukeland University Hospital, and high-resolution studies on protein or enzyme structure by the research group of José María Valpuesta, PhD, with the Spanish National Centre for Biotechnology in Madrid.