Potentially Self-Tuning DBS Device Monitors Brain Activity of Daily Life
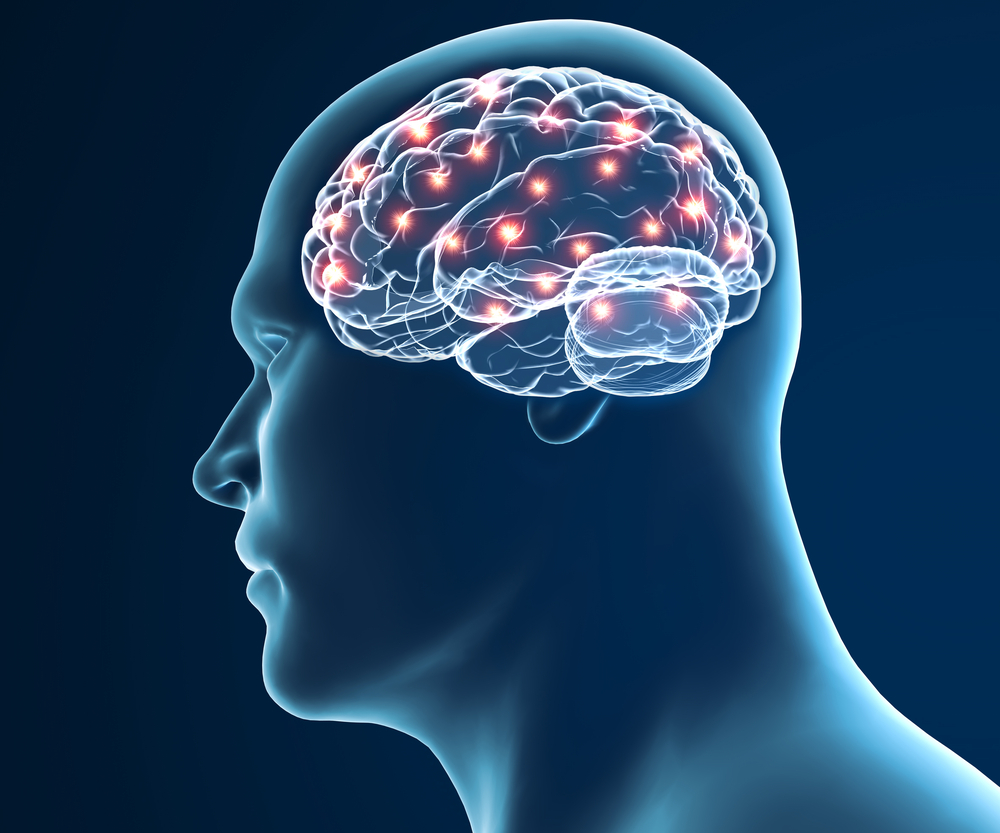
An experimental deep brain stimulation (DBS) system can continuously monitor brain activity in Parkinson’s disease patients as they go about their daily activities, with the information it produces used to adjust stimulation delivered by an implant device, the research team that developed it reported.
“This is really the first example of wirelessly recording deep and surface human brain activity for an extended period of time in the participants’ home environment,” Kari Ashmont, PhD, project manager for the National Institutes of Health’s Brain Research Through Advancing Innovative Neurotechnologies (BRAIN) Initiative, which funded the research, said in a press release.
“It is also the first demonstration of adaptive deep brain stimulation at home,” Ashmont added.
The findings, “Long-term wireless streaming of neural recordings for circuit discovery and adaptive stimulation in individuals with Parkinson’s disease,” were published in the journal Nature Biotechnology.
DBS is an established, surgical Parkinson’s treatment that involves implanting thin wires at specific regions of the brain to deliver continuous electrical signals generated by a battery-operated neurostimulator. These electrical signals are expected to help control Parkinson’s-associated abnormal brain activity, easing disease symptoms.
Adjustments in signal intensity are typically done manually by clinicians in response to changes in symptoms.
In 2018, however, a research team led by Philip Starr, MD, PhD, with the University of California, San Francisco, developed a new DBS device that delivered electrical stimulation to Parkinson’s patients only when needed, based on recorded brain activity.
Collected brain signals were fed to a computer program embedded in the device, which determined when to stimulate the brain, rather than provide continuous stimulation. This type of adaptive DBS system — one that responds to brain activity — is expected to be a safer alternative, as too much stimulation can cause uncontrollable involuntary movements.
Starr and his team, working with colleagues at other institutions, now report adding several features to the implanted technology to create the “first device that allows for continuous and direct wireless recording of the entire brain signal over many hours,” he said.
“That means we are able to perform whole brain recording over a long period of time while people are going about their daily lives,” Starr added.
This type of recording more accurately captures Parkinson’s brain activity patterns than do standard, short-term recordings in clinical settings, which are largely a snapshot of patients’ brain activity at precise moments.
As such, the new device may help to validate current brain activity patterns associated with Parkinson’s symptoms during ordinary daily activities.
“If you ever hope to use in-hospital recordings to modify a disease state through adaptive stimulation, you must show that they are also valid in the real world,” Starr said.
Another advantage of long-term recording is that it allows the identification of specific changes in brain activity, which can serve as biomarkers when they precede the onset of movement problems in individual patients.
Ro’ee Gilron, PhD, the study’s first author and a postdoctoral student in Starr’s lab, noted that this has the potential to allow a level of personalized DBS treatment that was previously impossible.
“Because we are able to build a biomarker library for each patient, we can now program each DBS unit according to a patient’s individual needs,” including “personalized stimulation programs that adapt as the patient’s needs change throughout the day,” Gilron said.
The potential for personalized stimulation was tested in five people with Parkinson’s disease, followed up for up to 15 months (about 1.5 years) after implantation of the new DBS device.
Brain activity data was collected through thin wires placed over the motor cortex and basal ganglia — two brain regions involved in movement control — and streamed to a pocket-sized device worn by a patient. Collected information was then transferred to a tablet through a wireless connection, and uploaded to a secure cloud.
Over 2,600 hours of patients’ brain activity data were paired with behavioral data from wearable monitors, to identify electrical patterns of inadequate or excessive movement for each patient.
“We validated individual-specific neurophysiological biomarkers during normal daily activities and used those patterns for adaptive deep brain stimulation,” the researchers wrote.
Notably, given that this system allows data to be monitored and analyzed without being in direct contact with a physician, it may also help reduce treatment burden for patients who live a distance from a clinic, and allow for patient monitoring while maintaining social distance during the current COVID-19 pandemic.
Patients can still “receive ‘over the air’ updates for their devices and telehealth visits from their neurologists as they manage increasingly complex DBS devices,” Gilron said.
This new technology marks a significant step toward better understanding not only neurological disorders, but also of the links between brain activity and behavior in general, Ashmont said.
However, extended — and potentially daylong — brain monitoring can raise ethical concerns, particularly regarding privacy.
“Although we are not at the point where we can distinguish specific normal behaviors from brain activity recording, [privacy] is an absolutely legitimate concern,” Starr said, adding that participants were told to “feel free to remove their wearable devices and to turn off their brain recordings whenever they engage in activities they would like to keep private.”
To help develop ethical guidelines and protocols for future projects, participants were invited to join a BRAIN Initiative’s neuroethics project looking to identify ethical concerns about the new device. Patients who chose not to go ahead with the stimulation study were also interviewed about their decision.