Neurons in Cell Cultures Form Complicated Networks, Study Shows
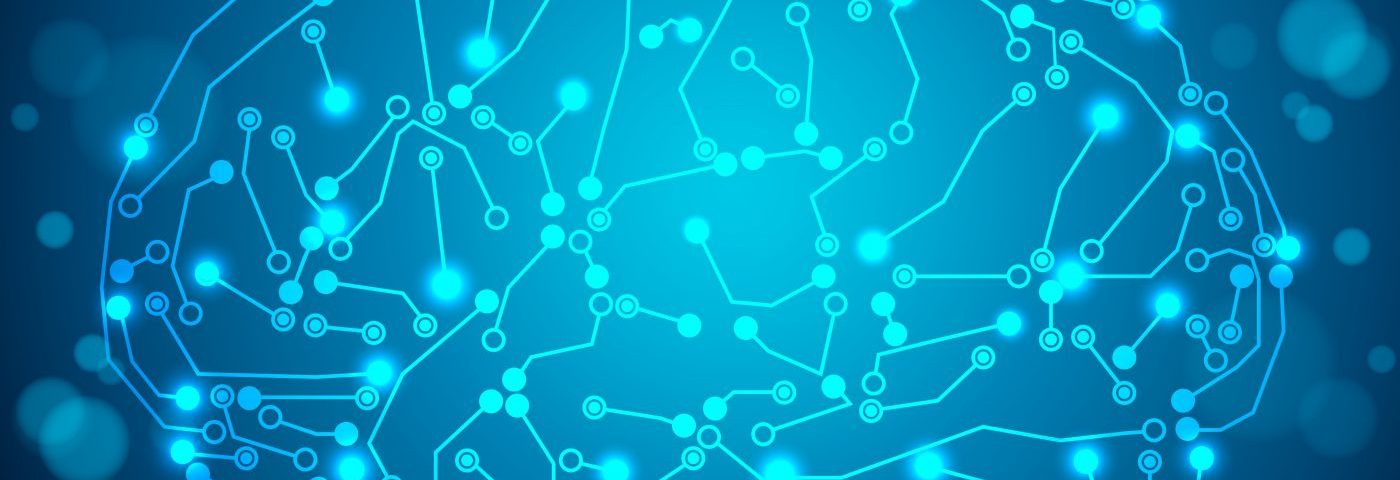
Rodent neurons (nerve cells) in cell cultures form complex interaction networks that seem designed to maximize information processing, but are distinct from many well-understood mathematical models, new research suggests.
Understanding how these networks form and interact within the brain could have applications in understanding neurological diseases like Parkinson’s and brain tumors. The results also may help inform the development of better computers.
The findings were published in Scientific Reports, in the study, “Network science characteristics of brain-derived neuronal cultures deciphered from quantitative phase imaging data.”
Neurons are cells in the nervous system that are able to communicate with each other by means of electrical and chemical signals. These cells form complicated communication networks — where one neuron will signal to one or more others, which signal to still others. The cross-talk between these networks gives rise to cognition and other neurological processes. Understanding exactly how these networks form and work is an ongoing area of investigation.
In cultures — grown in dishes under laboratory conditions — neurons are known to form complex networks, which are thought to mimic, at least to some degree, how these connections form in living organisms.
In the new study, researchers took advantage of new technology to examine the development of cultures of mouse and rat neurons. They used quantitative phase imagining to determine, in very precise detail, which neurons formed connections with each other over the course of the neural culture’s development.
“In this work, we investigated the mathematical properties of brain-derived neuronal culture networks and brain-derived neuronal culture cluster networks … which provides a way to analyze the spontaneous evolution of the neuronal cultures in the early stages,” the researchers wrote.
The researchers determined that, particularly at early time points in culture development, neurons tend to display assortative behavior — that is, they tend to connect to other neurons that have a similar number of connections. For instance, a neuron that already is connected to 11 other neurons is likely to form its 12th connection with another neuron that also has 11 other connections.
The researchers also noted that neurons tended to be more interconnected if they were in close physical proximity to each other.
Further mathematical analyses showed that the neurons tended to form networks that were optimized for information flow and for robustness. For example, the researchers found that, as the cultures developed, the neurons’ closeness centrality increased. Put very simply, this mathematical measurement indicates that, in more developed cultures, any given neuron’s message would have to be passed along by fewer of its fellows to get to its intended recipient neuron.
Conversely, betweenness centrality decreased as cultures matured. This is a measurement of the likelihood that a given neuron is necessary for getting a message between any other two neurons. So, a decrease in betweenness centrality means that the network as a whole is more robust, since deleting any single neuron would be less likely to impair more widespread communication.
“We observed that the brain’s networks have an extraordinary capacity to minimize latency, maximize throughput and maximize robustness while doing all of those in a distributed manner (without a central manager or coordinator),” study co-author Paul Bogdan, PhD, a professor at USC Viterbi School of Engineering, said in a press release.
The researchers examined whether the networks formed by the neurons mirrored well-known mathematical models for complex networks, such as the random regular and spatial scale-free models. However, the mathematical models generally were distinct from the observed behavior of the neurons.
“The neurons tend to form communities with very different topological structures than the well-known [models],” the researchers wrote.
These findings could have applications in diseases like Parkinson’s, where brain connections are disrupted. They also may be useful for identifying other diseases in which brain cells behave abnormally, such as brain cancer.
Of course, such applications will require additional research: “Future studies should characterize and distinguish between healthy and unhealthy behavior (e.g., glioblastoma/brain tumor) of neurons,” the researchers wrote.
Better understanding how connections form in the brain may aid in the development of better computational and artificial intelligence systems.
“Having this level of accuracy can give us a clearer picture of the inner workings of biological brains and how we can potentially replicate those in artificial brains,” Bogdan said.