Nerve Cell Signals That Guide Movement Are Complex and Dynamic, Study Finds
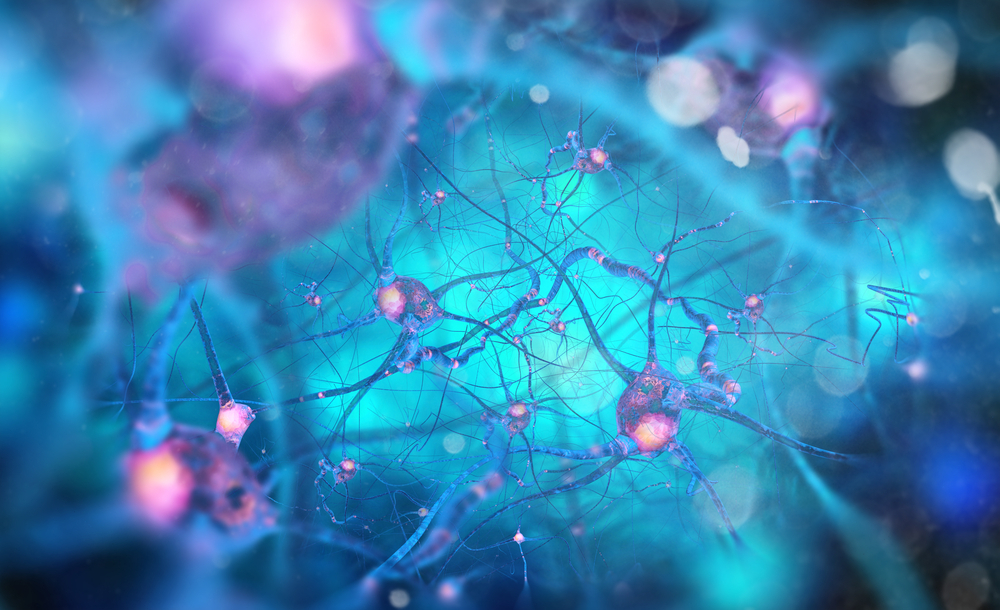
Rate hypothesis, a theory that Parkinson’s results from an imbalance in brain signals telling the body to start and stop moving, may involve “start” and “stop” signals that are more complex and intricate than initially thought, Stanford researchers report.
And, the researchers suggest, the loss of that complexity and structure might factor in the development of Parkinson’s disease.
Their study, “Diametric neural ensemble dynamics in parkinsonian and dyskinetic states,” was published in the journal Nature.
Parkinson’s is marked by the loss of dopamine neurons in a brain region called the substantia nigra, which is responsible for reward and movement, including the brain circuits that initiate and terminate movement.
“The idea was there was too much ‘stop’ and not enough ‘go,’ and that’s why there’s difficulty with movement,” Mark Schnitzer, an associate professor of biology and applied physics and the study’s lead author, said in a Stanford university news article by Nathan Collins.
Testing the rate hypothesis, however, has proven to be difficult, as the nerve cells controlling these stop and go signals are tightly interconnected. To assess that “start” neurons were suppressed and “stop” neurons hyperactive, as the hypothesis suggests, researchers need to track each type of neuron — and their activities — individually.
They used mice genetically engineered to have “stop” and “start” neurons that lit up in green whenever they were active.
The researchers then compared nerve cells dynamics in these mice under three conditions: a healthy condition, a condition that mimics Parkinson’s disease (using a neurotoxin to damage dopamine neurons in the substantia nigra), and that same Parkinson’s-like condition but in mice also treated with L-dopa (levodopa), a common patient medication.
Tiny microscopes allowed the team to look into mice brains and track the green flashes showing when “start” and “stop” neurons were active.
The results were surprising and contrary to what the rate hypothesis suggests: namely, that all neurons in the “start” and “stop” pathways light up at once. Rather, the researchers saw clusters of neurons firing in an activity-specific manner. For example, in healthy mice, a cluster of “start” neurons would turn on (become green) when a mouse began to turn left, while “stop” neurons would light up after that mouse finished grooming its tail.
In mice mimicking Parkinson’s disease, researchers saw a decrease in “start” neuron activity, as the rate hypothesis predicts. But those in the “stop” pathway were suppressing all kinds of movements at once, instead of particular movements like “stop grooming” or “stop turning left.”
Treatment with L-dopa restored both “stop” and “start” neuronal activity, but that structure came undone if the dose was too high: in these conditions, mice exhibited more activity in the “start” pathway, leading to random and uncoordinated movements similar to dyskinesia — the involuntary muscle movements that are a common side effect of L-dopa treatment in patients.
Overall, these findings reveal that both neuron activity rates and levels are affected in Parkinson’s disease and potentially other diseases sharing the same mechanisms, such as Huntington’s, Tourette’s syndrome, chronic pain and schizophrenia.
Importantly, when researchers compared L-dopa to two other less effective dopamine receptor agonists, only L-dopa was capable of fully restoring activity in neurons that control movement. This, the researchers suggested, means that scans of brain activity in patients might one day help to determine the effectiveness of a medication.
“What we may have here is a new way for testing and screening new drugs by looking directly at neural circuit activity,” Schnitzer said in the article.