Light Used to Control Brain Activity, Receptors Linked to Parkinson’s
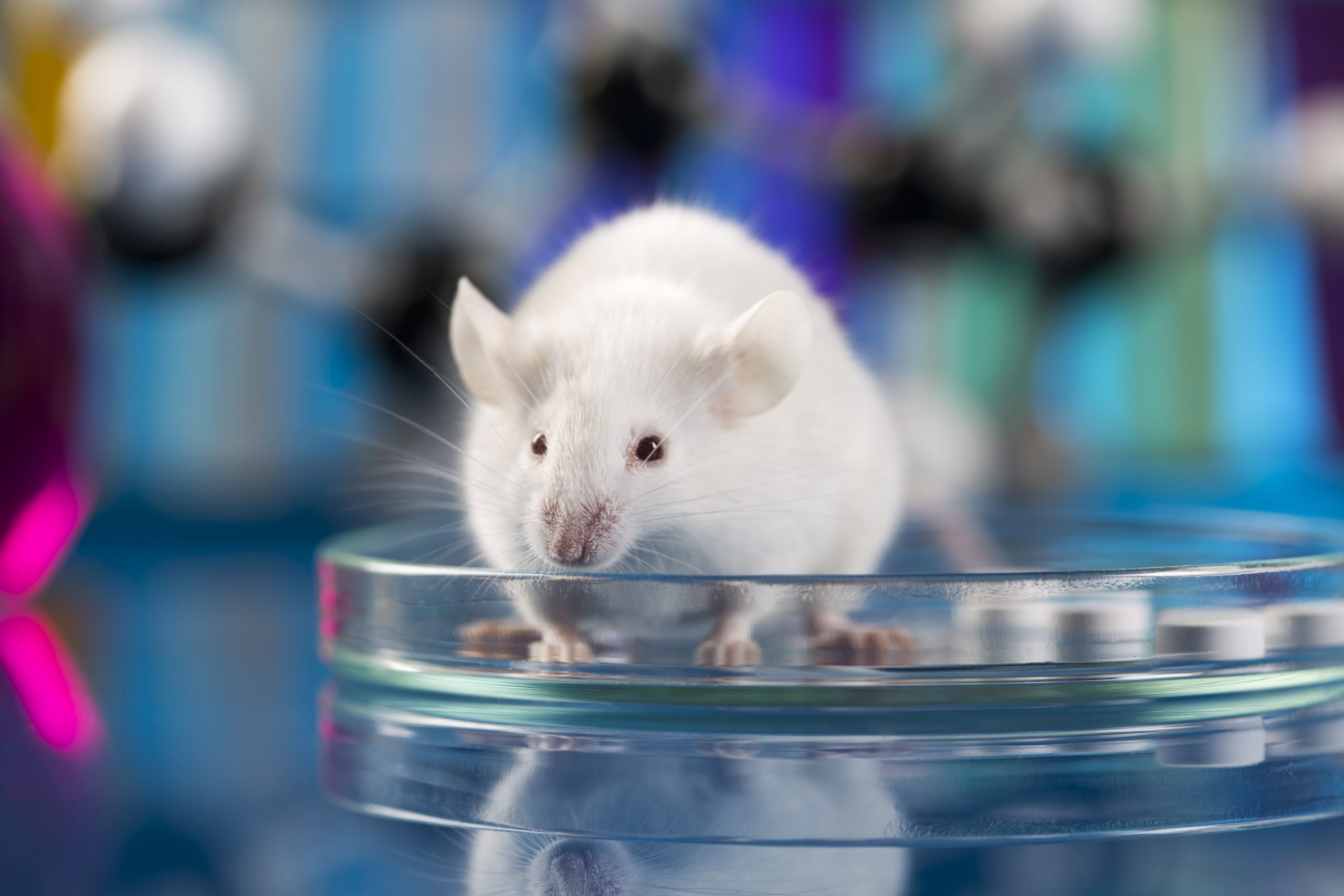
Exposing a molecule called PAI to light altered its shape, triggering an interaction with protein receptors — ones also associated with Parkinson’s disease — that control brain function, a study in mice demonstrated.
The findings support the development of light-sensitive methods to understand the underlying impact of Parkinson’s on the brain.
The researchers said this data also may lead to potential brain stimulation techniques or effective therapies.
The study, “Control of Brain State Transitions with a Photoswitchable Muscarinic Agonist,” was published in the journal Advanced Science.
In Parkinson’s disease, nerve cells or neurons in the brain — some of which produce the chemical dopamine — begin to malfunction or die. Because dopamine acts as a neurotransmitter, passing signals between neurons, it is vital for sending messages from the brain to control muscle movement and coordination throughout a person’s body.
A lack of dopamine leads to the characteristic motor symptoms seen in Parkinson’s patients.
Besides motor impairments, most people with the neurodegenerative disease will develop non-motor symptoms, such as changes in memory, language, or thinking, as well as mood disorders and sleep problems. Thus, the impact of neuron loss in Parkinson’s affects a wide range of brain functions.
Dopamine neurons themselves are regulated by proteins called muscarinic receptors, which are found in neurons throughout the central nervous system, composed of the brain and spinal cord. These receptors are known to modulate brain functions such as attention, learning, and memory, as well as sensory and motor functions.
They are stimulated by binding the neurotransmitter known as acetylcholine or ACh. The M2 type of the muscarinic receptor, called mAChR, is thought to be involved in neurodegenerative diseases such as Parkinson’s and Alzheimer’s. It has been seen as a possible therapeutic target to treat these disorders.
mAChRs also drive changes in different brain states, such as going from sleep to being awake. These states are associated with certain brain wave (oscillations) patterns with specific wave frequencies that can synchronize depending on the brain state. For example, deep sleep is associated with large, slow brain waves that are synchronized, whereas a waking state is linked to higher-frequency waves that are more out of sync (desynchronized).
Of note, brainwaves are produced by electrical pulses from neurons communicating with each other. They are divided into different bandwidths that change according to what an individual is doing and feeling.
The selective stimulation of mAChRs to modulate different brain states is an essential tool to learn about brain function and a better understanding of how diseases such as Parkinson’s affect brain function. This may lead to more accurate neuroscience research and the development of brain stimulation techniques or effective therapies.
“The control of neuronal activity in the brain is key to perform both basic and applied research, and to develop safe and accurate techniques to perform therapeutic brain interventions in clinical neurology,” Fabio Riefolo, PhD, of the Barcelona Institute for Science and Technology, in Spain, and the study’s co-first author, said in a press release.
In this study, Riefolo, along with Institute colleagues, in collaboration with scientists at the nearby August Pi i Sunyer Biomedical Research Institute (IDIBAPS), also in Spain, tested the ability of a molecule that activates M2 mAChRs called PAI to modulate different brain states.
The PAI molecule can have two distinct shapes: trans and cis. The transition between these two shapes can be achieved by exposing PAI to light. Notably, the trans form is a more potent activator of M2 receptors than cis. Thus, the direct activation of these receptors by light using PAI was used to investigate changes in brain states.
The team first applied different concentrations of trans-PAI and cis-PAI to thin slices of brain tissue isolated from ferrets to measure brain activity. In particular, they measured brain waves called slow oscillations, which are a hallmark of brain activity during sleep or when a person is under anesthesia. Pre-treatment slow oscillation patterns were recorded as a control.
Both trans-PAI and cis-PAI significantly modulated slow oscillations brain wave patterns in a dose-dependent manner. Of note, brain wave patterns result from repeated periods of high neuron activity — called Up-state — followed by near silence, known as Down-state.
As expected, the cis-PAI activation was weaker than trans-PAI, and they also showed a difference in the resulting brain wave frequencies of slow oscillations as well as the neuron firing (activity) rate during the Up-state. From these results, the team chose PAI concentrations to modulate slow oscillation waves in subsequent experiments.
Next, the team found that applying cis-PAI alone to brain slices did not impact the neuron firing rate but did evoke specific brain wave frequencies, rising from 0.53 cycles per second (Hz) to 1.04 Hz. Direct exposure to white light led to an increase in wave frequency, from 0.53 to 1.68 Hz, and a significant decrease in the Up-state neuron firing rate. “These differences are in agreement with PAI photoconversion to the active form (trans),” the researchers wrote.
As a proof-of-concept, the team aimed to control brain states with PAI and light in vivo, or in a living organism. Deep anesthesia was induced in healthy mice to reproduce the slow-wave sleep state. Part of the skull bone was removed, and an electrode was inserted into the brain, which also supplied PAI and UV light. As a control, the sleep-like slow oscillation patterns were recorded pre-treatment.
The brain wave frequency was not significantly altered by the administration of cis-PAI (0.58 Hz), and it caused only a slight increase in the neuron firing rate during the Up-states. Exposure to white light triggered a significant increase in brain wave frequency, from 0.60 to 0.73 Hz, while the neuron firing rate during Up-states was decreased.
“This light-dependent increase in oscillatory [wave] frequency activity of PAI in vivo is in partial agreement with the in vitro [in the laboratory] results observed in ferret slices, which also displayed a reduction in [firing rate],” the researchers said.
A final experiment in brain slices created an increase in wave frequency with trans-PAI. However, posteriorly applying cis-PAI to the same brain tissue led to the return of control-like wave activity, “demonstrating that the process is pharmacologically reversible,” they added.
This was the first study to look at these processes, the investigators said.
“A method for directly manipulating neural activity and brain rhythms with light-controlled drugs is demonstrated,” the team wrote.
“Thus, remote control of brain waves based on the photopharmacological manipulation of … muscarinic receptors may reveal the complex 3D molecular signaling underlying brain states and their transitions, in order to link them with cognition and behavior,” they wrote.
The scientists said this study’s findings will “open the way” for further neuroscience research and “to develop future brain therapies and stimulation.”
“The photocontrol of endogenous receptors and their functions in the central nervous system, such as the transition between different brain states, is an achievement for neuromodulation technologies,” said Almudena Barbero-Castillo, PhD, of IDIBAPS, the study’s co-first author.