Automated Process Grows 3D Organoids – ‘Mini-brains’ – to Test Potential Therapies
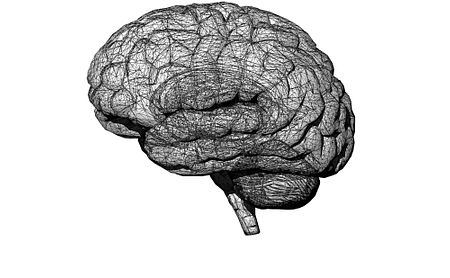
A fully automated process for growing three-dimensional (3D) midbrain-like organoids — a “mini-organ” model of the brain — has opened the door for the simultaneous testing of thousands of new potential Parkinson’s disease treatments.
The midbrain, the topmost part of the brainstem, contains approximately 75% of the dopamine-producing (dopaminergic) neurons, which are lost over the course of Parkinson’s. It’s also the site of the substantia nigra, a dopaminergic neuron-dense structure that controls movement.
In a proof-of-concept study, scientists at the Max Planck Institute for Molecular Biomedicine in Münster, Germany, showed how robotic lab technology could automate the entire experimental workflow, from cell culture to data analysis, pointing the way toward mass-producing midbrain and other organoids. These “mini-brain” models could be used for large-scale research into possible Parkinson’s therapies.
Their study, “A fully automated high-throughput workflow for 3D-based chemical screening in human midbrain organoids,” was published in eLife Sciences.
Complex 3D cell structures, organoids behave more similarly to real organs, making them a more attractive means of evaluating biological processes and screening compounds.
“Organoids are more complex, can more fully mimic the characteristic physiological properties of the tissue, and thus offer several functional advantages over traditional models,” Jan Bruder, PhD, the study’s principal investigator, said in a press release.
It can be difficult, however, to grow large numbers of organoids and ensure that the cells within them communicate with each other in biologically realistic ways. Without the ability to test compounds in large numbers of organoids, researchers can be left unsure of whether a potential therapy actually works or if it was simply tested in a “good” organoid.
The Max Planck team overcame these challenges by designing a robotic process that grew thousands of midbrain organoids from human cells. Each “mini-brain” grown this way was nearly identical to the others, giving confidence that individual variation would not skew experimental results.
In keeping with the mini-brain analogy, the midbrain organoids showed synchronized neural activity, similar to that seen in the brain itself.
One of the team’s innovations was to grow their midbrain models from small molecule neural precursor cells (smNPCs), rather than from induced pluripotent stem cells (iPSCs), as is more often done.
Taken from adult skin or blood samples, iPSCs can grow into any cell type and replicate indefinitely, making them a powerful and versatile research tool. Growing them into desired cell types and then larger, more complex structures, however, is a time-consuming task. smNPCs represent a later time point in nerve cell development, which the scientists said helped to further their research.
“Instead of growing midbrain neurons from iPS cells over one or two months, we used smNPCs,” says Henrik Renner, PhD, the study’s lead author. “This allowed us to obtain dopamine-producing nerve cells within two weeks.”
The same procedure that the robot uses to seed the cells that become organoids can be used to screen compounds in them, making even experimentation an automated step. Through this system, the effects of therapies can be assessed at both the whole-organoid and single-cell levels.
Finally, by including data analysis in their protocol, the team ensured that the entire procedure of growing midbrain organoids, performing experiments on them, and analyzing the results could be performed efficiently, reproducibly, and at scale.
The new technology addresses a need within the scientific community, the researchers noted. To find even a single therapeutic molecule, up to hundreds of thousands may have to be tested. Doing this in a scalable, reproducible, automated, and highly sensitive way promises to accelerate drug discovery while lowering a considerable part of the cost of early research.
“We hope that our automation approach can contribute to establishing a next generation of cellular 3D in vitro disease models that allow unbiased, quantitative, high-throughput access to human tissue-surrogates in a dish,” the researchers wrote.