Magnetic Invention Represents Mechanical Method of Stimulating Neurons Remotely
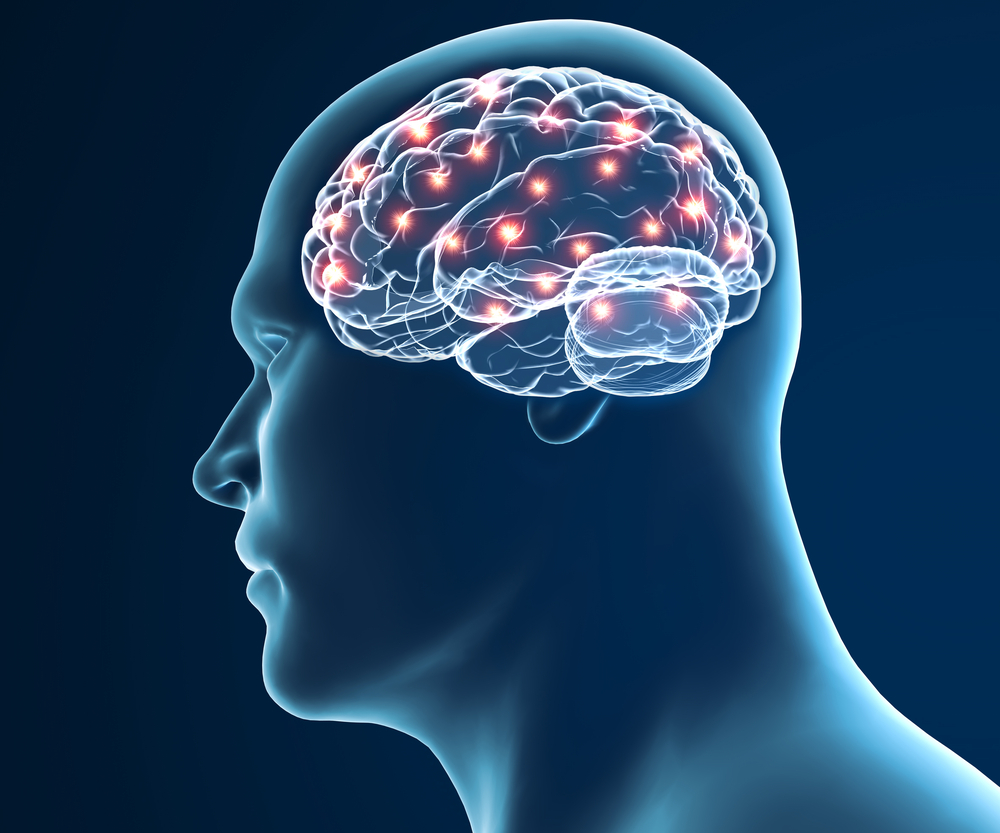
Researchers have developed tiny magnetic discs that open the possibility of a contact-free mechanical way to remotely stimulate brain nerve cells in people with neurological conditions, including Parkinson’s disease.
The study describing that finding, “Magnetic Vortex Nanodiscs Enable Remote Magnetomechanical Neural Stimulation,” was published in ACS Nano.
Brain nerve cells (neurons) respond to chemical and electronic stimuli, and therapies directed at malfunctioning neurons accordingly consist of either chemicals — medications — or electrical currents, such as in the case of deep brain stimulation.
However, neurons also respond to mechanical stimulation, such as pressure and vibration. Mechanical responses are more difficult to study, however, because there is no easily controllable way to mechanically manipulate neurons in a living creature.
Researchers at the Massachusetts Institute of Technology (MIT) and colleagues, invented a way to remotely control magnetic nanoparticles capable of exerting small amounts of pressure on individual cells. This technique might make it possible to advance new types of therapeutic treatments, similar to electrically-based therapies, such as neurostimulation, that have been used to treat Parkinson’s and other neurological disorders.
Whereas current systems rely upon invasive wires, the MIT system would theoretically require only an initial injection of nanoparticles, after which therapy would be controlled by an external magnetic field, making it contact-free.
The nanoparticles consist of miniscule discs (98−226 nanometers), roughly a hundredth the size of the neurons they are meant to stimulate. Despite their small individual size, they can be injected in enough quantity to collectively activate a cell’s pressure receptors. The discs remain inert until activated by a particular magnetic field, at which point, they move to exert force on a cell.
Polina Anikeeva, PhD, the study’s lead author, called finding a material that could provide enough force with only a moderate magnetic activation “a very hard problem,” in a news story on MIT’s website.
So, Anikeeva and her colleagues had to invent a new kind of magnetic disc to achieve their goal.
When a magnetic metal alloy that would have been able to provide the necessary forces for neurostimulation proved both prohibitively expensive and incompatible with biological tissue, the team found a way to create its own material by converting hematite, which could form the required discs, into magnetite, which has the required magnetic properties and is benign to the body. (Hematite and magnetite are both iron ore minerals.)
To get their magnets to work, the team had to alter a key physical property.
Magnets have a property called “spin,” which determines how they act within a magnetic field. Normally, a given magnet will have a single well-defined spin. The MIT team’s discs are special, in that they contain multiple simultaneous spins, such that without an applied field, they behave as if they weren’t magnets at all, making them exceptionally stable in solutions. When a magnetic field is turned on within their range, their internal spins align, allowing researchers to use the field to manipulate them.
To test their discs, researchers incubated them with dorsal root ganglia explants, which contain sensory neurons that express several receptors that can sense such mechanical forces. After eight days of incubation, these cells remained viable and the extent of the neuronal response was directly correlated with the volume of the discs and the strength of the magnetic field.
Of note, these magnetic discs drove cellular signaling by activating ion channels involved in sensing mechanical signals and not by directly changing the permeability of the cell membrane.
Anikeeva drew attention to the highly interdisciplinary nature of this project, which drew heavily from the fields of chemistry, electromagnetism, and the biology of neurostimulation.
This technology is currently in its infancy and much remains to be done, to actually bring it into a clinical setting. It is an important first step, however, in that the invention opens up the real possibility of bioelectronic medicine, without the need for drugs or electrodes.
“This means,” said Anikeeva, “that anywhere in the nervous system where cells are sensitive to mechanical forces, and that’s essentially any organ, we can now modulate the function of that organ.”